Ion the Prize: The 2019 Nobel Prize in Chemistry

The awarding of the 2019 Nobel Prize in Chemistry to John B. Goodenough, M. Stanley Whittingham, and Akira Yoshino for inventing the lithium-ion battery speaks to the centrality of this device in contemporary society. This battery stores more energy and releases it more quickly than any other rechargeable alternative, and that quality has transformed the way we work and live.
Practically all mobile phone and wireless handheld devices use the battery, and it has long been the unsung hero of the information technology revolution, every bit as important as the microprocessor in enabling mobile computing. In the hands of Tesla, lithium-ion power disrupted the auto sector, changing the public perception of the electric car as stodgy, underpowered, and short-legged. In a very real sense, we live in what the journalist Seth Fletcher termed a lithium economy.
Like so many stories about innovation, the history of the lithium-ion battery is characterized by contributions made over many years by many people (far more than the Nobel committee has acknowledged). These contributions have been conditioned by a changing economic and social dynamic, which can be boiled down to four things. The first is interdisciplinarity. Goodenough, a professor at the University of Texas at Austin, isn't a chemist, and his Nobel triumph in that field must surely amuse him. Trained as a theoretical physicist specializing in solid-state physics, he has described his career as one in which he designed experiments for chemists to execute. The second factor is serendipity. As more than one observer has noted, luck is the constant companion of successful science. A third element is public policy, essential for nurturing disruptive ideas the market would otherwise suppress in the name of short-term gain. And the fourth is internationalism.
Their work consisted of ransacking the periodic table of elements to find combinations of materials that produced a cheap, durable, and safe battery.In each of these respects, the story of how Goodenough, Whittingham, and Yoshino invented lithium-ion power illustrates how much science-based innovation has changed over the years. It begs comparison to another famous Nobel trio, the Bell Labs team of Walter Brattain, John Bardeen, and William Shockley, who in 1947 invented the world's first working transistor, the ancestor of the silicon-based semiconductor and microprocessor.
Goodenough roughly parallels Bardeen as the respective theorist of the group, but the similarities largely end there. Where the Bell Labs boys worked together as a team over a few years in a single institution that has become a virtual metonym of American innovation, Goodenough, Whittingham, and Yoshino essentially worked independently in a series of institutions in different countries over a much longer period of time, dating back to the early 1950s.
Basically, their work consisted of ransacking the periodic table of elements to find combinations of materials that produced a cheap, durable, and safe battery. The reason lithium rechargeables are so potent is that they are packed with highly reactive and flammable materials, especially lithium metal oxides. Getting the balance right, as Goodenough once told me, was the difference between having a useful battery and an improvised explosive device.
The origin story of the lithium-ion battery does not begin with batteries at all but with computers. It traces to Goodenough's experiments with metal oxides as a young physicist at MIT's Lincoln Laboratory, where in the early 1950s he joined the team working on the magnetic memory for Whirlwind, the digital computer that controlled the radars of America's first air defense early-warning system. It was the heyday of Big Science, a time when undirected research was thought to be the secret sauce for technological innovation and economic growth. When Whirlwind concluded in the late 1950s, Goodenough stayed on at Lincoln Labs, where he was allowed to pursue fundamental research on metal oxides on his own terms for a decade, all on the taxpayers' dime.
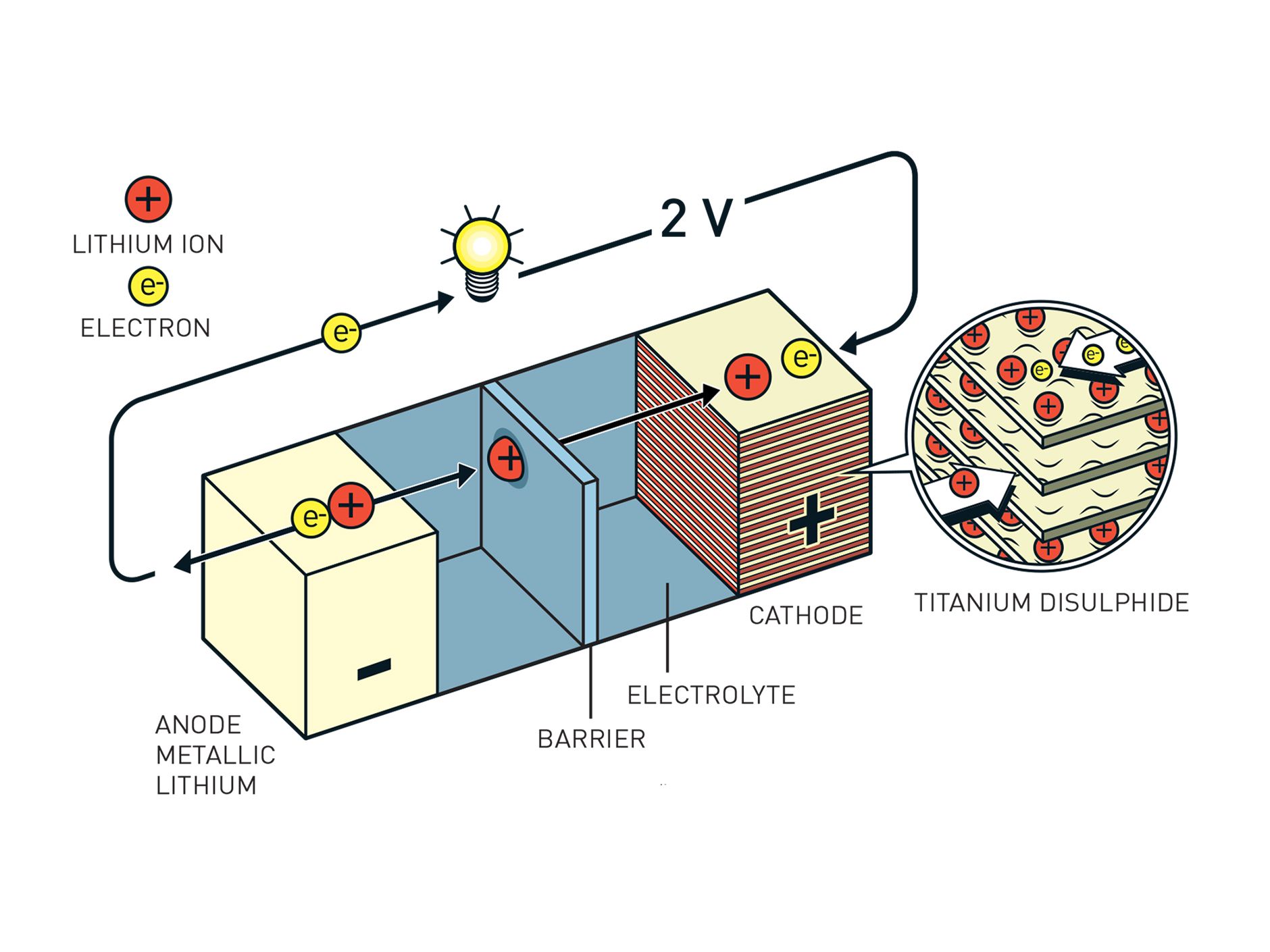
Whittingham's battery was the first to use "intercalation," the reversible storage of lithium ions in the layered structure of the cathode. Illustration: Johan Jarnestad/The Royal Swedish Academy of Sciences
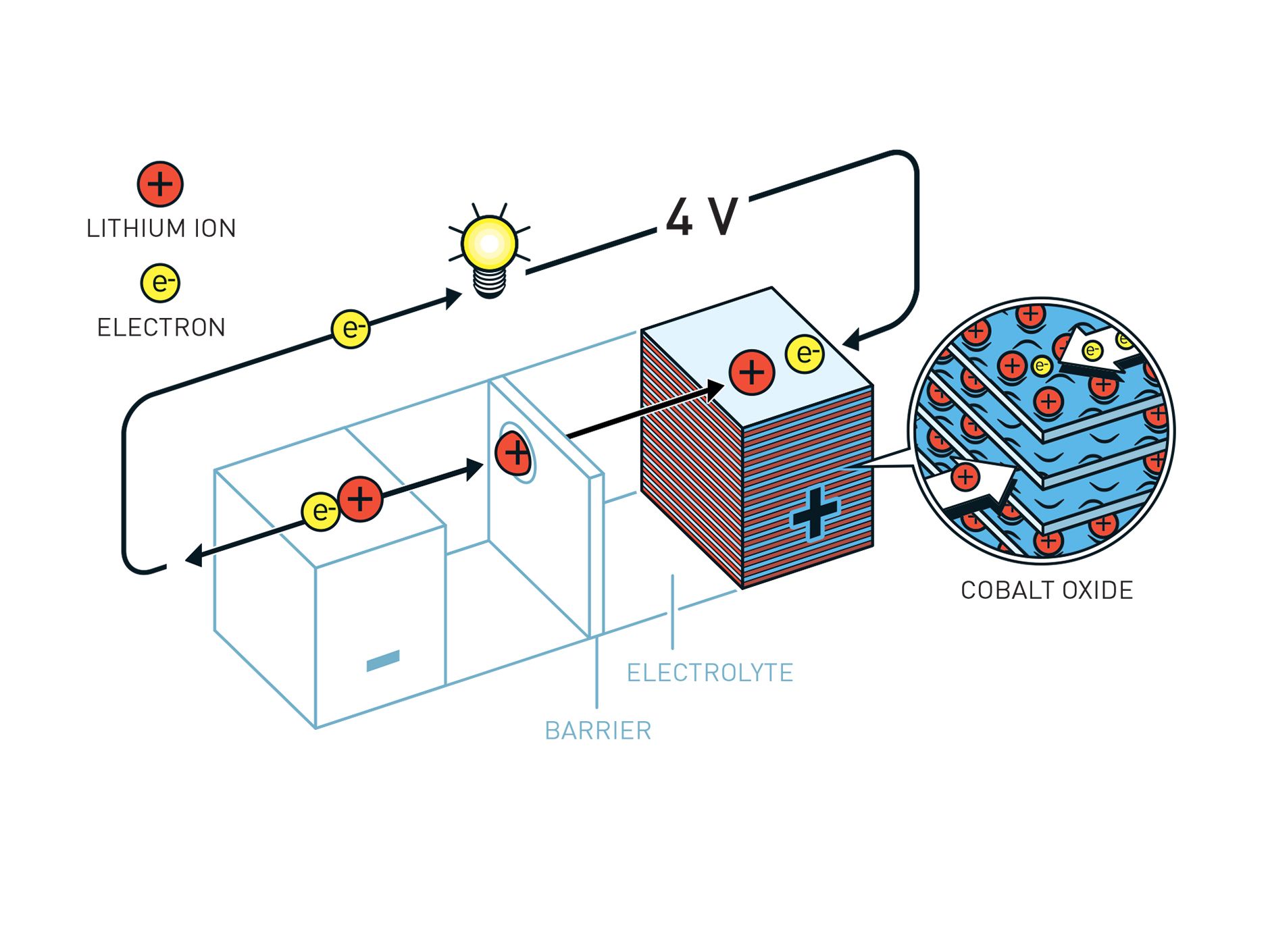
Goodenough's battery used different materials in the cathode and so was much more stable. Illustration: Johan Jarnestad/The Royal Swedish Academy of Sciences
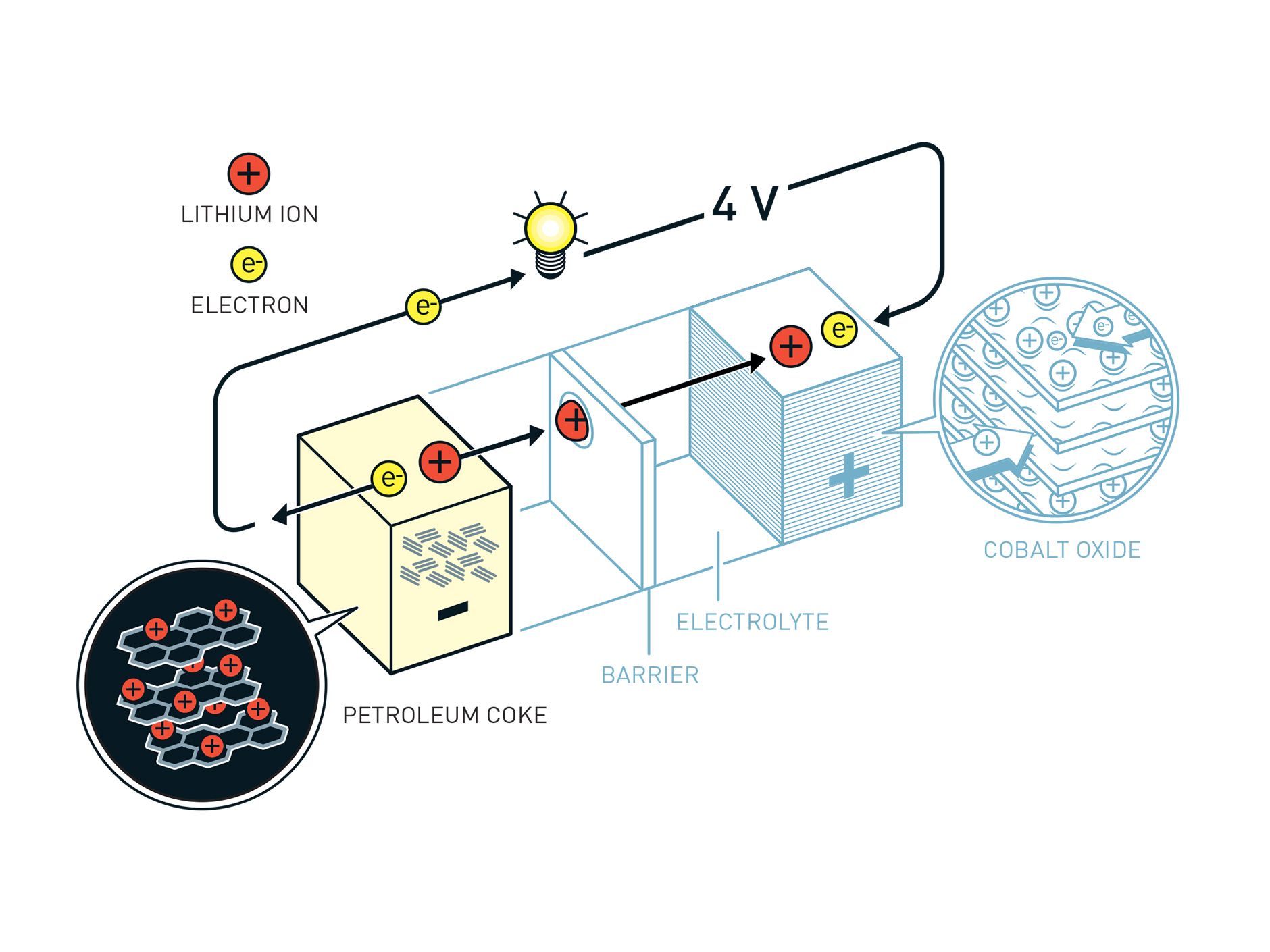
Yoshino's battery was the first to demonstrate a safe anode made of carbon. Illustration: Johan Jarnestad/The Royal Swedish Academy of Sciences
Previous NextFast forward to the 1970s. It might be a surprise to learn that an oil company funded development of the first true lithium-ion battery, but that's exactly what happened during the energy crisis. Faced with the possibility that automakers might be forced to build electric cars, oil interests wanted to hedge their bets and be in line to supply the power sources. It was in the employ of Exxon that the British-American chemist Whittingham, now a professor at the State University of New York at Binghamton, devised a chemistry based on titanium disulfide that he used to demonstrate "intercalation," the reversible storage of ions in a layered structure, the basic operating principle of a rechargeable lithium battery.
There was a problem: Whittingham had devoted his attention to the positive electrode, or cathode; the other electrode, the anode, he made of metallic lithium, which is highly reactive. Repeated charging and discharging induced an electrochemical reaction among these substances that turned the battery into a bomb.
"After Exxon blew up a couple labs, they got out of the advanced battery industry," recalled Goodenough.
Increasingly, U.S. policymakers wanted science to pay a quick technological dividend in service of pressing social and economic needs.Meanwhile, shifts in U.S. science policy dislodged Goodenough from his niche at Lincoln Labs. Rising civil rights and antiwar activism focused scrutiny on the Pentagon's involvement in academic science. In 1969 and 1971, Congress passed the Mansfield Amendments, ending military support of undirected academic research and scotching the biggest patron that university-based science had ever had. Moreover, U.S. science policymakers were becoming disenchanted with the core premise of basic undirected research. Increasingly, they wanted science to pay a quick technological dividend in service of pressing social and economic needs.
When the newly formed Department of Energy commandeered all of Goodenough's research as part of its reorganization of federal energy research and development in 1976, the physicist decamped to Oxford University, in England, where he took the chair of its inorganic chemistry laboratory. It was in these genteel environs that he and the Japanese physicist Koichi Mizushima developed the battery material that would alter the course of consumer electronics. Aware of Whittingham's experience with sulfides, Goodenough and Mizushima used layered metal oxides as cathode materials, settling on lithium cobalt oxide as the best balance between stability and energy.
But they still lacked a suitable safe anode. The trigger for that innovation came from consumer electronics. Although Japanese companies were largely frozen out of personal computing by the Wintel duopoly of Microsoft Windows and Intel semiconductors, they dominated pretty much everything else in consumer electronics in the 1980s and 1990s. Cheap and powerful microprocessors enabled Japanese designers to create a host of handheld devices, which in turn created a need for a new rechargeable that was up to the task of supplying these electron-thirsty applications with juice. In the mid-1980s, Sony's Energytec division began working on a plan to develop a safe anode for the lithium cobalt oxide cathode with a view to replacing the less capable nickel-cadmium battery. The solution was graphite, a stable and nonreactive material that facilitated safe recharging. This component was developed in good measure by Yoshino at Asahi Kasei chemical, but its commercialization also owed much to Sony researcher Yoshio Nishi. In 1991, Sony was first to market with a commercial lithium cobalt oxide battery, which became the standard rechargeable chemistry in notebooks, mobile phones, and eventually, computer phones.
In the 1990s, public policy, now in the form of environmental policy, reemerged as the impetus for perhaps the most portentous chapter of lithium power. California's Zero Emission Vehicle mandate of 1990 forced automakers to build electric cars and helped stimulate massive federal research in advanced batteries, including lithium-ion systems. But lithium found its way into electric cars only after mainstream automakers temporarily rolled back the mandate and recalled and destroyed their fleets of electric cars in the early 2000s, an act of folly that outraged enthusiasts and goaded a young electrical engineer named Martin Eberhard into an act of do-it-yourself retribution. If automakers were not going to deliver the clean cars that Californians demanded, he reasoned, why not build his own around a massive battery, the most powerful ever put into an electric car, using thousands of lithium cobalt oxide notebook batteries? An improbable plan, it became the basis of a startup that upended business as usual in automaking. Eberhard went on to found Tesla Motors with Marc Tarpenning, J.B. Straubel and Elon Musk, and its success as the only major company to manufacture exclusively all-battery electric vehicles, aided by generous state and federal subsidies, triggered a butterfly effect that saw mainstream automakers scrambling to get back into a space they once scorned.
The history of the lithium-ion battery shows that while Americans may no longer monopolize innovation and its rewards, the pros of global collaboration far outweigh the cons.In the story of the commercialization of the lithium-ion battery there are plenty of lessons for contemporary policymakers. Hardly anyone would dispute the centrality of basic science to technological innovation, but predicting the circumstances in which it will yield useful technology is a fool's game. It used to be that large, vertically integrated corporations like IBM, General Electric, and above all AT&T/Bell Labs did their own basic science, back in the day when they were so big and rich that they could afford to shell out for blue-sky thinking. Those days are long gone.
Among the reasons the information technology revolution took off in the 1970s were the efficiencies to be had in specialization. No one company did it all. Some, like Intel, manufactured components, while others like Apple and Qualcomm specialized in design; still others, like Dell, aimed at systems integration. Offshoring and outsourcing helped Silicon Valley generate fabulous wealth, and while policymakers and pundits have long fretted about what the disappearance of classic American idea factories like Bell Labs might mean for the future, innovation is a transnational phenomenon.
The history of the lithium-ion battery shows that while Americans may no longer monopolize innovation and its rewards, the pros of global collaboration far outweigh the cons. It also shows that when the market fails to deliver equitable outcomes, public policy can step into the breach. With its trade wars, nativism, and attempts to stop California from regulating its own air quality, the current U.S. administration would do well to keep that history in mind.
About the AuthorMatthew N. Eisler is a Strathclyde Chancellor's Fellow and lecturer in history at the University of Strathclyde, in Glasgow. His 2012 book Overpotential: Fuel Cells, Futurism, and the Making of a Power Panacea (Rutgers) explored the long road to commercialize the fuel cell.