Rerouting Intention And Sensation In Paralyzed Patients
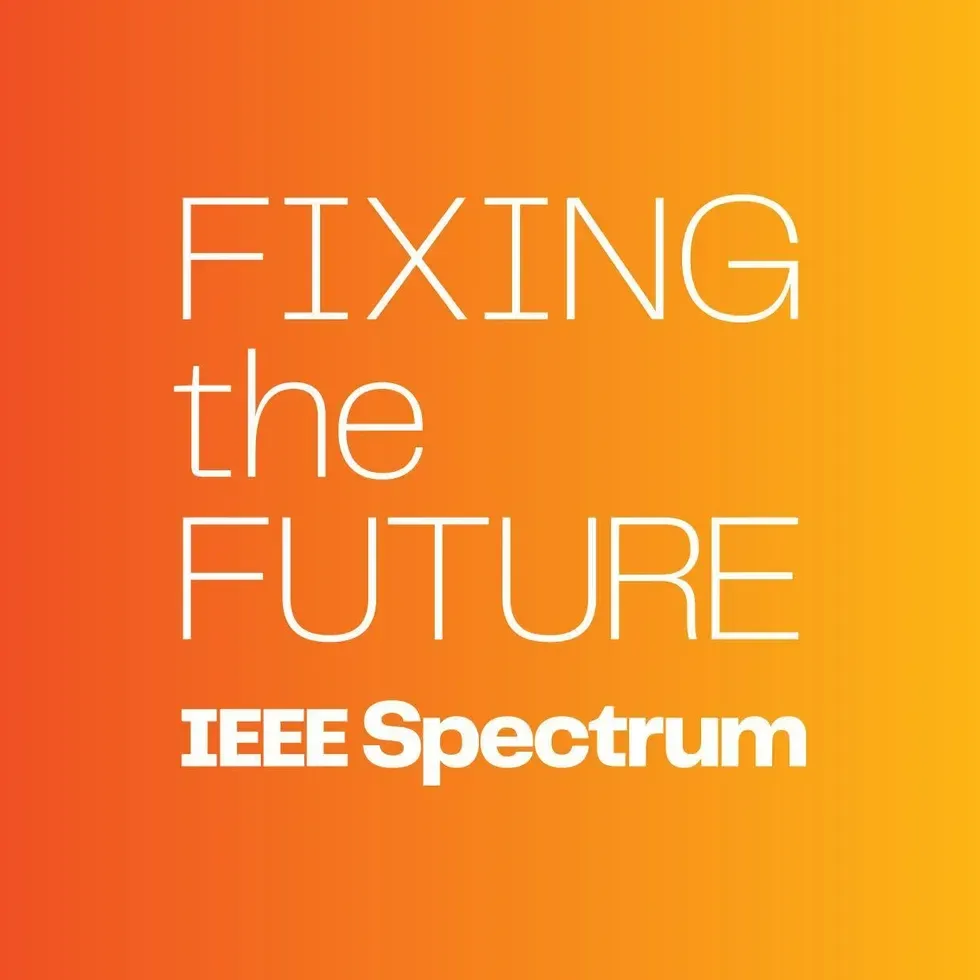
Transcript
Eliza Strickland: Paralysis used to be thought of as a permanent condition, but over the past two decades, engineers have begun to find workarounds. They're building on a new understanding of the electric code used by the nervous system. I'm Eliza Strickland, a guest host for IEEE Spectrum's Fixing the Future podcast. Today I'm talking with Chad Bouton, who's at the forefront of this electrifying field of research. Chad, welcome to the program, and can you please introduce yourself to our listeners?
Chad Bouton: Yes, thanks so much, Eliza, for having me. And my name is Chad. I'm at the Northwell Health Feinstein Institute for Medical research.
Strickland: And can you tell me a bit about the patient population that you're working with? I believe these are people who had become paralyzed, and maybe you can tell us how that happened and the extent of their paralysis.
Bouton: Absolutely. Absolutely. In fact, we work with folks that have been paralyzed either from a traumatic injury, stroke, or even a brain injury. And there's over 100 million people worldwide that are living with paralysis. And so it's a very devastating and important condition, and we are working to restore not only movement, but we're making efforts to restore sensation as well, which is often not the focus and certainly should be.
Strickland: So these are people who typically don't have much movement below the head, below the neck?
Bouton: So we have focused on tetraplegia or quadriplegia because, obviously, it's extremely important and it is very difficult to achieve independence in our daily lives if you don't have the use of your hands in addition to not being able to move around and walk. And it surprisingly accounts for about half of the cases of spinal cord injury, even slightly more than half. And it used to be thought of as something that was a more rare condition, but with car accidents and diving accidents, it's a prominent and critical condition that we need to really address. And there's no cure currently for paralysis. No easy solution. No simple fix at this point.
Strickland: And from your experiences working with these people, what kind of capabilities would they like to get back if possible?
Bouton: Well, individuals with paralysis would like to really regain independence. I've had patients and study participants comment on that and really ask for advances in technology that would give them that independence. I'll speak to some of the things we're doing in the lab, but folks often ask, Could we take this home or take it outside the lab?" And we're certainly working to do that as well. But the goal is to be more independent, ask for help less, be able to achieve functional abilities to do even things that we might consider just basic necessities, feeding, grooming, and even some of the personal aspects, being able to hold someone's hand and to feel that person's hand or a loved one's hand. Those are the things that we're really targeting and working hard to address.
Strickland: Yeah, I thought it's really interesting that your group is focused on hands. There are other groups that are working on letting people walk again, but the hands feel like a very obviously important target too.
Bouton: Yeah, absolutely. And in fact, there's been studies and widespread surveys on this topic, and folks that are living with tetraplegia or quadriplegia prioritize or say their top desire is to move their hands again. And if you step back and think about it for a second, it makes sense because we rely on our hands so much. And even losing one hand, say from a stroke, can be devastating and very disruptive to our lives.
Strickland: Yeah, let's go over the basics of electrophysiology for listeners who don't have a background in that area. I love this field. It has such a long history that goes back to the 1780s when Luigi Galvani touched an exposed nerve of a dead frog with a scalpel that had an electric charge and saw the frog's leg kick.
Bouton: Yes.
Strickland: Can you explain how the nervous system uses electricity?
Bouton: Yes, absolutely. So it's an electrochemical phenomenon. And of course, it involves neurotransmitters as well. When a neuron fires, as we say, that's an electrical impulse. It only lasts a very brief moment, less than a thousandths of a second. But basically, there's a polarization of the neuron itself and charges that are passing through ion channels. So what does this mean? Well, it's kind of like in a computer where you have zeros and ones. For a brief moment, that cell has changed from, let's say, a zero to a one, and it is firing or having this impulse that represents that binary one. And what's so neat about it is that the firing rate, so basically how often those impulses are happening or how fast they're happening, carries information. And then, of course, which neurons or nerve fibers carry the information or which ones are firing is what we call spatial encoding. So you have temporal encoding and spatial encoding. Those together can carry a tremendous amount of information or can mean different things, whether it's a motor event where there's a need to activate certain muscles in the hand or the fingers or the legs and any muscle throughout the body. And we also have sensory information that gets encoded by the same approach. And so information can pass from the brain to the body and from the body back to the brain, and we have these two-way information highways all throughout our central and peripheral nervous system. I call it often the most complex control system in nature, and we're still trying to understand it.
Strickland: Yeah, so for a person with tetraplegia, these electrical messages from the brain are essentially not getting through. The highway is blocked, right?
Bouton: That's right. Absolutely. And so let's walk through that scenario. So now, someone who's had a car accident or a diving accident, often the highest level of stress occurs at the base of the neck, and we call that C5, so it's the cervical, a fifth vertebra there. Often that cord gets damaged because the vertebra itself, which normally would protect that cord, unfortunately, it gets fractured and can then slip or slide and can actually crush or damage the cord itself. So then what is often misunderstood is that you don't get a simple complete shutdown. You get damage and certain levels of damage or amounts of damage. And what can happen is someone can become paralyzed but lose sensation as well along with motor capability. It's not going to be the same for everyone. There's different levels of it. But usually, there's damage, and signals are able to get through but often very attenuated, very weak. And so I'll talk through some of the approaches we're taking now to boost, if you will, those signals and try to enhance those signals. The good news is that we're finding more and more that those signals are there and can be boosted or enhanced, which is very, very exciting because it's opening new doors to new therapies that we're developing.
Strickland: Yeah, I love that you call your system the neural bypass, which is very evocative. You can imagine picking up the signals in the brain, getting around the blockage, and sending the information onto the muscles. So maybe we can talk about the first part of that first. How do you get the information from the brain?
Bouton: Well, yes, the neural bypass, so it's funny because that phrase was used very briefly back in the 70s. And then it kind of went away and I think really because it wasn't possible with technology at that time. But then in the early 2000s, we started to really explore this concept and use that phrase again and say, if we can put a microelectrode array in the brain, which we did back around 2005, 2006, and a number of colleagues and various team members kind of looked at that and said, yes, we can record from the brain. We can even stimulate the brain. But we said, why couldn't we take that information, reroute it, as you say, around an injury or even a damaged part of the nervous system or the brain itself and create this neural bypass, and then reinsert the signals or link those signals directly to muscle stimulation? And that was what we called the one-way bypass, neural bypass. And why couldn't we do that and restore movement? And so we attempted to do that and were thankfully successful in 2014. In fact, we had enrolled a young man named Ian Burkhart. His name, of course, became public, and he was the first paralyzed individual to regain movement using a brain implant that formed this neural bypass, this one-way or unidirectional neural bypass. And it was very, very exciting, and he was able to do some pretty amazing things with this approach. And in fact, I still remember when he first drank from a glass on his own. He reached out, opened his fingers using the bypass, which he hadn't been able to do for four years since his accident, and he was able to open his hand by himself without help, pick up a glass, bring it to his lips, and be able to just take a drink. It was really quite a moment, and the entire team and myself were very moved and we thought we're really taking an important step forward here.
Strickland: Ian Burkhart also played Guitar Hero if I remember right. Is that correct?
Bouton: Yeah, so another very, very exciting moment was when we explored the idea of rhythmic movements in the hand. So I'll do a little experiment here. We'll do it even though this is a podcast, but we can all do this experiment. If you hold up one hand-- and you should try this, Eliza. Okay, so hold up, say, either left or right. Now take your other hand and drum your fingers against the palm of your hand and go very, very fast. Okay, now stop, and now try to reverse directions. Okay. And is it awkward and harder? Okay, so now pay attention to which way was the fastest, what we would call, quote, natural" way for you. Was it pinky to index or index to pinky?
Strickland: Pinky to index was really easy for me. The other way was almost impossible.
Bouton: Okay, well, you're what we call the normal group. So the 85 percent of population does the faster, more natural direction from pinky to index. Only 15 percent of the population goes from index to pinky. And the question is, why in the world is there a wiring, if you will, or a natural direction? And we looked at rhythmic movements. As we looked at the electrode array and the signals we were recording, we could see there was a group or an ensemble of neurons that were firing when we are thinking about rhythmic movements, say just wiggling a finger. And then the other, there's a totally different group when you actually try to do a static movement of that finger. You're trying to press it and hold that finger in a certain position. So we thought, let's see if we can decipher these different groups. And then we linked those signals back to neuromuscular stimulators that we had developed, and we then asked the question, could Ian or others move the fingers in a more dynamic way? And we published another paper on this, but he was able to dynamically move his fingers and then also statically move those, and he could then play Guitar Hero just by thinking about different static or sustained movements and holding a note, let's say, in the guitar or dynamically doing riffs. And we have videos and whatnot online. But it was really amazing to deepen our understanding but also to allow, again, a little more independence, allow someone to do something fun, a little bit more recreational too.
Strickland: Sure, sure. So Ian was using implanted electrodes to get his brain signals. Can you walk us through the different approaches in plants versus wearables?
Bouton: Yes, actually, there are a number of ways of tapping into the nervous system and specifically into the brain. And a more recent approach we've been taking is to use a minimally invasive procedure to place a very thin electrode. It's called a stereo electroencephalogram-type electrode, an SEEG. And these are used routinely at our location and a number of locations around the world for mapping the brain in epilepsy patients. But now we ask the question, well, could we use these electrodes to record and stimulate in the motor and sensory area? And we just recently this past year did both, and our findings were quite striking. We were able to not only decode individual finger movements with this different type of electrode and approach, but we were also able to stimulate in primary sensory cortex actually down in the central sulcus. That's right between your motor and sensory area. And on the wall of the sulcus on the sensory side, we were able to stimulate and elicit highly focal percepts at the fingertips. And this has been a challenge with different electrodes, like the kind of electrodes that I was previously talking about, which were placed on the surface of the brain, not down into the sulcus. So this has allowed us to answer new questions and is also opening up a door to a minimally invasive approach that could be extremely effective in trying to restore even finer movements of the human hand and also sensations. You have to know that you can't button your shirt without tactile feedback, and getting that feedback at the tips of the fingers is so important for fine motor tasks and dexterous hand movement, which is one of the goals of our lab and center.
Strickland: Yeah, I wanted to ask about this idea of the two-way bypass. So in this idea, you have sensors on your fingers or on your hand, and those are sending information to electrodes that are conveying it to the brain?
Bouton: That's absolutely right. With the fingertips and the thin membrane sensors that we've developed, we can pick up not only the pressure level that the fingertips but also even directional information. So in other words, when we pick up, say, a cup, I have one here on my desk, and I'm picking this cup up. There's a downward, what we call shear force that's pushing the skin down towards the floor. And this is additional information the brain receives so that we know, oh, we're picking something up that has some weight to it. And you don't even realize you're doing this, but there's a circuit, a relatively complex circuit that involves interneurons in the spinal cord that tightens that grip naturally. You don't, again, realize you're doing it. Just a little subtle increase in your grasp. And so when we want to create a bidirectional or a two-way neural bypass, we have to use that information from the sensors, we have to route that back into our computer, we have to decode or decipher that information. That part is straightforward from the sensors, but then how do you encode that information so the brain will interpret that as, oh, I feel not only some kind of sensation at my fingertips, but what's the level of that sensation?
And we just, again, last year, were able to show that we can encode the different levels of pressure or force felt, and the participants have reported very accurately what those levels are. And then once the computer understands and interprets that and then starts to send signals back to another set of what we call microstimulators that stimulates the brain, again, with the right firing rate or frequency, then the challenge still remains to make that feel natural. Right now, people still report it's a bit of a slightly artificial sensation sometimes, or they feel like, I feel this pressure in different levels, but it's a little bit electrical or even mechanical like a vibration. But it is still extremely useful, and we're still refining that. But now what you've done is you've started to close the loop, right? Not only can signals from the brain be interpreted and sent to stimulation devices for muscle activation, we can also pick up the sensation, the tactile sensation, send it back into the brain, and now we have a fully closed loop or a bidirectional bypass.
Strickland: So when you're sending commands to muscles to have the hand do some movement, how much do we understand the neural code that makes one finger move versus another one?
Bouton: Yeah, that's a great question. So we surprisingly understand a fair amount on that after many years and many groups looking at this. We now understand that we can change the firing rate, and we can change how fast we're stimulating or how fast we need to stimulate that muscle to get a certain contraction level. Recording this signal, understanding the signal from the motor cortex in the brain and how that translates to a different level of contraction, we also understand much better now. Even understanding if it should be a static movement or a dynamic movement, I spoke a little bit to that. I think what's hard, that we're still trying to understand, is synergistic movements, when you want to activate multiple fingers together and do a pinch grasp or you want to do something more intricate. There have been studies where people have tried to understand the signal when someone flips a quarter between the fingers, you've seen this trick, or a drum stick when you're spinning it around and manipulating it and transferring it from one pair of fingers to another. Those super complex movements involve motor and sensory networks working together very, very, very closely. And so even if you're, say, listening in or eavesdropping in on the motor cortex, you only really have half the picture. You only have half the story.
And so one of the things we're going to be looking at, and we now have FDA clearance to do this, is to record in both motor and sensory and then to be able to stimulate in the sensory area of the brain. But by recording in both motor and sensory, we can start to look more deeply into this question of, well, how are those networks communicating with each other? How do we further decode or decipher that information? I have someone in my lab, Dr. Sadegh Ebrahimi, who did his graduate work at Stanford and his postdoc work there, he looked at the question of how do different areas of the brain communicate and pass these massive amounts of information back and forth, and how are they connected, and how does this information flow? He is going to be looking at that question along with, can we use reinforcement learning techniques to further refine our decoding and more importantly our encoding and how we stimulate and how we even stimulate the muscles and get all of these networks working together?
Strickland: And for the electrodes that are controlling movement, are those a wearable system that people can just have on their arm?
Bouton: Yes, we're very excited to announce that we're now developing wearable versions of the neuromuscular stimulation technology, and our hopes are to make this available outside the lab in the next year or two. What we have done is we've developed very thin, flexible electrode arrays that have been ruggedized and encapsulated in a silicone material. And there are literally over 200 electrodes now that we have in these patches, and they're able to precisely stimulate different muscles. But what's so fascinating is that by using the right electrical waveforms, and we have been optimizing these for a number of years, but in the right electrode array design, turns out we can isolate individual finger movements very accurately. We can even get the pinky to move in very unique ways and the thumb in multiple directions. And with this approach and it also being wireless, people can, with this being lightweight and thin, they can actually wear it under their clothes and folks can use it out and about, outside the lab, in their homes. And so we're really looking forward to accelerating this.
And you can link this wearable technology either to a brain-computer interface, which is what we've been talking a lot about, or there's even a stand-alone mode where it uses the inertial sensing of what we call body language or basically body movements. These would be the residual movements that individuals are able to do even after their injury. It might be shoulder movement or lifting their arm. Often, in a C5-level injury, the biceps are spared, thankfully, and one can lift their arm and lift their shoulders. So folks can reach, but they can't open and use their hand. But with this technology, we infer what they want to do. If they're reaching for a cup of water, we can infer, ah, they're reaching with a certain trajectory, and we use our machine learning or AI algorithms to detect, even before the hand gets to the target, we know, ah, they're trying to reach and do what we call a power grasp or a cylindrical grasp. And we start to stimulate the muscles to help them finish that movement that they can't otherwise do on their own. And this will not allow, say, playing Guitar Hero, but it is allowing folks to do very basic types of actions like picking up a cup or feeding themselves. We have a video of someone picking up a granola bar and a participant that fed himself for the first time. And that was also really an incredible moment because really achieving that independence is what we're trying to do at the end of the day.
Strickland: Yeah, let's talk a little bit about commercialization. I imagine it's a very different story when you're talking about brain implants versus noninvasive devices. So where are you in that pathway?
Bouton: Yeah, so you're absolutely right. There's a big difference between those two pathways. I spent many years commercializing technologies. And when you take them out of the lab and try to get through what we call the valley of death, it's a tough road. And so what we decided to do is carve out the technology from the lab that was more mature and had a more direct regulatory path. We have been working closely with the FDA on this. We formed a company called Neuvotion, and Neuvotion is solely focused on taking the noninvasive versions of the technology and making those available to users and those that really can benefit from this technology. But the brain-computer interface itself is going to take a little bit longer in terms of the regulatory pathway. Thankfully, the FDA has now issued as of last year a guidance document, which is always a first step and a very important step, available. And this is a moment in time where it is no longer a question of whether we will have brain-computer interfaces for patients, but it's now just a question of when.
Strickland: Before we wrap up, I wanted to ask you about another very different approach to helping people with tetraplegia. So some researchers are using brain-computer interface technology to read out intentions from the brain, but then sending those messages to robotic limbs instead of the person's own limbs. Can you talk about the tradeoffs, the challenges, and the advantages of each approach?
Bouton: Absolutely. So the idea of using a brain-computer interface to interface with a robotic arm was and is an important step forward in understanding the nervous system and movement and even sensation. But the comment I heard from a number of participants through the years is that at the end of the day, they would like to be able to move their own arm and feel, of course, with their own hands. And so we have really been focused on that problem. However, it does bring in some additional challenges. Not only is a biological arm more complex and more difficult to control and you have fatigue, muscle fatigue, and things like this to deal with, but also, there's another complication in the brain. So when we reach out for something, we pick up a cup, I talked earlier about the nervous system reacts to the weight of the cup and different things happen. Well, there's another issue, too, when you stimulate in the sensory area and you cause a percept. Someone says, Okay, I feel kind of pressure on my fingertips." Well, the sensory cortex is right next door to the motor cortex primary, S1 and M1 as they're called. And so you have all these interconnections, a huge number of interconnections.
And so we hypothesize and we have some evidence already on this is that when you stimulate and you start to encode and put information or you're writing into the brain, if you will, well, guess what? When you're on the read side and you're reading from the motor cortex, because of all those interconnections, you're going to cause changes in what we call modulation. You're going to see changes in patterns. This is going to make the decoding algorithms more difficult to architect. We predicted this would happen when Ian became the first person to move their hand and to be able to pronate his arm. We predicted that during the transfer of objects, there might be difficulties and changes in the modulation and would affect the decoding algorithms. And indeed that did happen. So we believe as we close the loop on this bidirectional neural bypass, we're going to run into similar challenges and changes in modulation, and we're going to have to adapt to that. So we're also working on adaptive decoding. And there's been some great work in this area, but with actually reanimating or enabling movement and sensation in the human arm itself and the human hand itself, we believe we're in for some additional challenges. But we're up for it, and we are very excited to move into that space of this year.
Strickland: Well, Chad, thank you so much for joining us on the Fixing the Future podcast. I really appreciate your time today.
Bouton: Absolutely. Glad to do it, and thanks so much for talking with me.
Strickland: Today on Fixing the Future, we were talking with Chad Bouton about a neural bypass to help people with paralysis move again. I'm Eliza Strickland for IEEE Spectrum, and I hope you'll join us next time.