No Propeller? No Problem. This Blimp Flies on Buoyancy Alone

On a cold March night last year in Portsmouth, England, an entirely new type of aircraft flew for the first time, along a dimly lit 120-meter corridor in a cavernous building once used to build minesweepers for the Royal Navy.
This is the Phoenix, an uncrewed blimp that has no engines but propels itself forward by varying its buoyancy and its orientation. The prototype measures 15 meters in length, 10.5 meters in wingspan, and when fully loaded weighs 150 kilograms (330 pounds). It flew over the full length of the building, each flight requiring it to undulate up and down about five times.
Flying in this strange way has advantages. For one, it demands very little energy, allowing the craft to be used for long-duration missions. Also, it dispenses with whirring rotors and compressor blades and violent exhaust streams-all potentially dangerous to people or objects on the ground and even in the air. Finally, it's cool: an airship that moves like a sea creature.
This propulsion concept has been around since 1864, when a patent for the technique, as applied to an airship, was granted to one Solomon Andrews, of New Jersey (U.S. Patent 43,449). Andrews called the ship the Aereon, and he proposed that it use hydrogen for lift, to make the ship ascend. The ship could then vent some of the hydrogen to reduce its buoyancy, allowing it to descend. A return to lighter-than-air buoyancy would then be achieved by discarding ballast carried aloft in a gondola suspended beneath the airship.
The pilot would control the ship's attitude by walking along the length of the gondola. Walking to the front moved the center of gravity ahead of the center of buoyancy, making the nose of the airship pitch down; walking to the back would make the nose pitch up.

Andrews suggested that these two methods could be used in conjunction to propel the airship in a sinusoidal flight path. Raising the nose in ascent and lowering it in descent causes the combination of aerodynamic force with either buoyancy (when lighter than air) or with weight (when heavier than air) to have a vector component along the flight path. That component provides the thrust except at the top and bottom of the flight path, where momentum alone carries it through. The flight tests we performed were at walking pace, so the aerodynamic forces would have been very small. There will always be a component of either buoyancy or weight along the flight path.
The method Andrews describes in his patent means that the flight had to end when the airship ran out of either hydrogen or ballast. Later, he built a second airship, which used cables to compress the gas or let it expand again, so that the airship could go up and down without having to jettison ballast. His approach was sound: The key to unlocking this idea and creating a useful aircraft is thus the ability to vary the buoyancy in a sustainable manner.
A variation on this mode of propulsion has been demonstrated successfully underwater, in remotely operated vehicles. Many of these gliders" vary the volume of water that they displace by using compressed air to expand and contract flexible bladders. Such gliders have been used as long-distance survey vehicles that surface periodically to upload the data they've collected. Because water is nearly 1,000 times as dense as air, these robot submarines needn't change the volume of the bladders very much to attain the necessary changes in buoyancy.
Aeronautical versions of this variable-buoyancy concept have been tried-the Physical Science Laboratory at New Mexico State University ran a demonstration project called Aerobody in the early 2000s-but all anyone could do was demonstrate that this odd form of propulsion works. Before now, nobody ever took advantage of the commercial possibilities that it offered for ultralong endurance applications.
The Phoenix project grew out of a small demonstration system developed by Athene Works, a British company that specializes in innovation and that's funded by the U.K. Ministry of Defense. That system was successful enough to interest Innovate UK, a government agency dedicated to testing new ideas, and the Aerospace Technology Institute, a government-funded body that promotes transformative technology in air transport. These two organizations put up half the 3.5 million budget for the Phoenix. The rest was supplied by four private companies, five universities, and three governmental organizations devoted to high-value manufacturing.
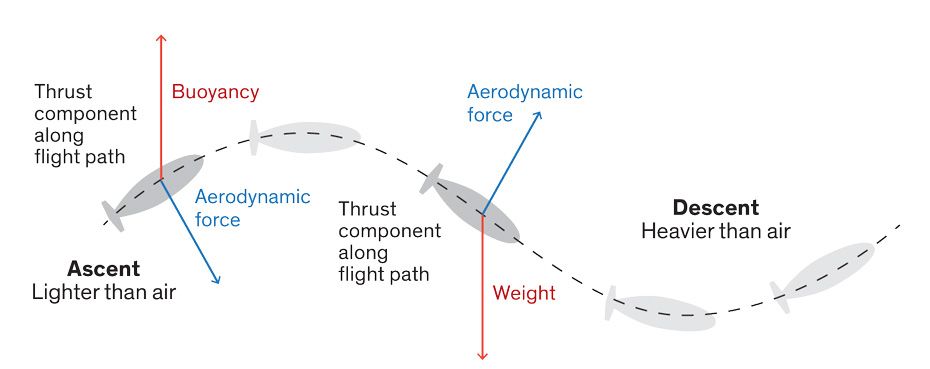

My colleagues and I had less than four years to develop many of the constituent technologies, most of which were bespoke solutions, and then build and test the new craft. A great number of organizations participated in the project, with the Centre for Process Innovation managing the overall collaboration. I served as the lead engineer.
For up-and-down motion, the aircraft takes in and compresses air into an internal lung," making itself heavier than air; then it releases that compressed air to again become lighter than air. Think of the aircraft as a creature that inhales and exhales as it propels itself forward.
The 15-meter-long fuselage, filled with helium to achieve buoyancy, has a teardrop shape, representing a compromise between a sphere (which would be the ideal shape for maximizing the volume of gas you can enclose with a given amount of material) and a long, thin needle (which would minimize drag). At the relatively low speeds such a craft can aspire to, it is enough that the teardrop be just streamlined enough to avoid eddy currents, which on a sphere would form when the boundary layer of air that lies next to the surface of the airship pulls away from it. With our teardrop, the only drag comes from the friction of the air as it flows smoothly over the surface.
The skin is made of Vectran[PDF], a fiber that's strong enough to withstand the internal pressure and sufficiently closely knit so that together with a thermoplastic polyurethane coating it can seal the helium in. The point was to be strong enough to maintain the right shape, even when the airship's internal bladder was inflating.
Whether ascending or descending, the aircraft must control its attitude. It therefore has wings with ailerons at the tips to control the aircraft's roll. At the back is a cross-shaped structure with a pair of horizontal stabilizers incorporating elevators to control how the airship pitches up or down, and a similar pair of vertical stabilizers with rudders to control how it yaws left or right. These flight surfaces have much in common with the wood, fabric, and wire parts in the pioneering airplanes of the early 20th century.
Two carbon-fiber spars span the wings, giving them strength. Airfoil-shaped ribs are distributed along the spars, each made up of foam sandwiched between carbon fiber. A thin skin wraps around this skeleton to give the wing its shape. We designed the horizontal and vertical tail sections to be identical to one another and to the outer panels of the wings. Thus, we were able to limit the types of parts, making the craft easier to construct and repair.
Sunny Side UpSolar electric power, backed up by batteries or fuel cells, can supply the day-to-day needs of a blimp flying in place, as a pseudosatellite. Here you can see the assembly and testing of the photoelectric system on the wings and horizontal tails [top]; the movement of the combined vertical and horizontal tail structure [top middle]; the inspection of the horizontal tail [bottom middle]; and the placement of the component in the Phoenix.




An onboard power system supplies the electricity needed to operate the pumps and valves used to inflate and deflate the inner bladder. It also energizes the various actuators needed to adjust the flight-control surfaces and keeps the craft's autonomous flight-control system functioning. A rechargeable lithium-ion battery with a capacity of 3 kilowatt-hours meets those requirements in darkness. During daylight hours, arrays of flexible solar cells (most of them on the upper surfaces of the wings, the rest on the upper surface of the horizontal tail) recharge that battery. We confirmed through ground tests outdoors in the sun that these solar cells could simultaneously power all of the aircraft's systems and recharge the battery in a reasonable amount of time, proving that the Phoenix could be entirely self-sufficient for energy.
We had envisaged also using a hydrogen fuel cell, but because of fire-safety requirements it wasn't quite ready for the indoor flight trials. We do plan to add this second power source later, for redundancy. Also, if we were to use hydrogen as the lift gas, the fuel cell could be used to replenish any hydrogen lost through the airship's skin.
So how well did the thing fly? For our tests, we programmed the autonomous flight-control system to follow a sinusoidal flight path by operating the valves and compressors connected to the internal bladder. In this respect, the flight-control system has more in common with a submarine's buoyancy controls than an airplane's flight controls.
We had to set strict altitude limits to avoid contact with the roof and floor of the building during our indoor test. In normal operation, the aircraft will be free to determine for itself the amplitude of its up-and-down motion and the length of each undulation to achieve the necessary velocity. Doing that will require some complex calculations and precisely executed commands-a far cry from the meandering backward and forward in a wicker gondola that Andrews did.
Although our experiments to date are merely testing a previously unproven concept, the Phoenix can now serve as the prototype for a commercially valuable aircraft. The next step is getting the Phoenix certified as airworthy. For that, it must pass flight trials outdoors. When we planned the project, this certification had a series of weight thresholds, with 150 kg being the upper limit for approval through the U.K. Civil Aviation Authority under a permit to fly." Had it been heavier, approval by the European Union Aviation Safety Agency would have been needed, and trying to obtain that was beyond our budget of both time and money. After the United Kingdom fully exits from the European Union, certification will be different.
Commercial applications for such an aircraft are not hard to imagine. A good example is as a high-altitude pseudosatellite, a craft that can be positioned at will to convey wireless signals to remote places. Existing aircraft designed to perform this role all need very big arrays of solar cells and large batteries, which add to both the weight and cost of the aircraft. Because the Phoenix needs only small arrays of solar cells on the wings and horizontal tail, it can be built for a tenth the cost of the solar e-planes that have been designed for this purpose. It is a cheap, almost disposable, alternative with a much higher ratio of payload to mass than that of alternative aircraft. And our designs for a much larger version of the Phoenix show that it should be feasible to lift a payload of 100 kg to an altitude of 20 kilometers.
We are now beginning to develop such a successor to the Phoenix. Perhaps you will see it one day, a dot in the sky, hanging motionless or languidly porpoising to a new position high above your head.
This article appears in the July 2020 print issue as This Blimp Flies on Buoyancy Alone."
About the AuthorAndrew Rae is a professor of engineering at the University of the Highlands and Islands, in Inverness, Scotland.