Goodbye, Centralized Power Grid. Hello, Autonomous Energy Grids

In the Colorado neighborhood of Basalt Vista, a microgrid allows 27 households to seamlessly share electricity when needed. So far, utility bills are about 85 percent lower than typical electric bills in the state. The local electrical utility, Holy Cross Energy, partnered with the National Renewable Energy Laboratory to implement this autonomous energy grid.
It's great to have neighbors you can depend on, whether you're borrowing a cup of sugar or you need someone to walk your dog while you're out of town. In the western Colorado neighborhood of Basalt Vista, the residents are even closer than most: They share their electricity. But unlike your neighbor with the sugar, the residents of Basalt Vista may not even know when they're being generous. The energy exchanges happen automatically, behind the scenes. What residents do know is how inexpensive, reliable, and renewable their electricity is.
The 27 smart homes in Basalt Vista, located about 290 kilometers west of Denver, are part of a pilot for an altogether new approach to the power grid. The entire neighborhood is interconnected through a microgrid that in turn connects to the main grid. Within each home, every smart appliance and energy resource-such as a storage battery, water heater, or solar photovoltaic (PV) system-is controlled to maximize energy efficiency.
On a larger scale, houses within the neighborhood can rapidly share power, creating reliable electricity for everyone-solar energy generated at one house can be used to charge the electric car next door. If a wildfire were to knock out power lines in the area, residents would still have electricity generated and stored within the neighborhood. From the spring through the fall, the PV systems can provide enough electricity and recharge the batteries for days at a time. In the dead of winter, with the heat running and snow on the solar panels, the backup power will last for about 2 hours.
In theory, power systems of any size could be covered in a patchwork of Basalt Vistas, layering regions and even an entire country in smart grids to automatically manage energy production and use across millions of controllable distributed energy resources. That concept underlies the autonomous energy grid (AEG), a vision for how the future of energy can be defined by resilience and efficiency.
The concept and core technology for the autonomous energy grid are being developed by our team at the National Renewable Energy Laboratory, in Golden, Colo. Since 2018, NREL and local utility Holy Cross Energy have been putting the concept into practice, starting with the construction of the first four houses in Basalt Vista. Each home has an 8-kilowatt rooftop PV system with lithium iron phosphate storage batteries, as well as energy-efficient, all-electric heating, cooling, water heaters, and appliances. All of those assets are monitored and can be controlled by the AEG. So far, average utility bills have been about 85 percent lower than typical electric bills for Colorado.

The all-electric homes in Colorado's Basalt Vista use smart controllers from Heila Technologies [right] to manage the photovoltaic panels, batteries, electric-vehicle charging, heating, and cooling. In the event of an outage on the regional grid, the neighborhood can still draw electricity from battery storage and rooftop solar.
AEGs will create at least as many benefits for utilities as they do for customers. With AEGs monitoring distributed energy resources like rooftop solar and household storage batteries, a utility's control room will become more like a highly automated air traffic control center. The result is that energy generated within an AEG is used more efficiently-it's either consumed immediately or stored. Over time, the operator will have to invest less in building, operating, and maintaining larger generators-including costly peaker" plants that are used only when demand is unusually high.
But can a network as large and complicated as a national power grid really operate in a decentralized, automated way? Our research says definitely yes. Projects like the one at Basalt Vista are helping us figure out our ideas about AEGs and demonstrate them in real-world settings, and thus are playing a crucial role in defining the future of the power grid. Here's how.
Today, grid operators must overcome two big problems. First, an ever-growing number of distributed energy resources are being connected to the grid. In the United States, for instance, residential solar installations are expected to grow approximately 8 percent per year through 2050, while household battery systems are estimated to hit almost 1.8 gigawatts by 2025, and around 18.7 million EVs could be on U.S. roads by 2030. With such anticipated growth, it's possible that a decade from now, most U.S. electricity customers could have a handful of controllable distributed energy resources in their homes. By that math, Pacific Gas & Electric Co.'s 4 million customers in the San Francisco Bay Area could have a total of some 20 million grid-tied systems that the utility would need to manage in order to reliably and economically operate its grid. That's in addition to maintaining the poles, wires, transformers, switches, and centralized power plants in its network.

The Basalt Vista autonomous energy grid uses a 900-megahertz radio antenna to communicate with Holy Cross Energy's dispatch center, about 50 kilometers away.
Because of the soaring number of grid-tied devices, operators will no longer be able to use centralized control in the not-so-distant future. Over a geographically dispersed network, the communication latencies alone make a centralized system impractical. Instead, operators will have to move to a system of distributed optimization and control.

The other problem operators face is that the grid is functioning under increasingly uncertain conditions, including fluctuating wind speeds, cloud cover, and unpredictable supply and demand. Therefore, the grid's optimal state varies every second and must be robustly determined in real time.
A centrally controlled grid can't handle this amount of coordination. That's where AEGs come in. The idea of an autonomous energy grid grew out of NREL's participation in a program called NODES (Network Optimized Distributed Energy Systems) sponsored by the U.S. Department of Energy's vanguard energy agency, ARPA-E. Our lab's contribution to NODES was to create algorithms for a model power grid made up entirely of distributed energy resources. Our algorithms had to factor in the limited computational capabilities of many customer devices (including rooftop solar, electric vehicles, batteries, smart-home appliances, and other loads) and yet still allow those devices to communicate and self-optimize. NODES, which wrapped up last year, was successful, but only as a framework for one cell"-that is, one community controlled by one AEG.
Our group decided to carry the NODES idea further: to extend the model to an entire grid and its many component cells, allowing the cells to communicate with one another in a hierarchical system. The generation, storage, and loads are controlled using cellular building blocks in a distributed hierarchy that optimizes both local operation and operation of the cell when it's interconnected to a larger grid.
In our model, each AEG consists of a network of energy generation, storage, and end-use technologies. In that sense, AEGs are very similar to microgrids, which are increasingly being deployed in the United States and elsewhere in the world. But an AEG is computationally more advanced, which allows its assets to cooperate in real time to match supply to demand on second-by-second timescales. Similar to an autonomous vehicle, in which the vehicle makes local decisions about how to move around, an AEG acts as a self-driving power system, one that decides how and when to move energy. The result is that an AEG runs at high efficiency and can quickly bounce back from outages, or even avoid an outage altogether. A power grid that consists entirely of AEGs could deftly address challenges at every level, from individual customers up to the transmission system.
To develop the idea, we had to start somewhere. Basalt Vista presented an excellent opportunity to bring the AEG concept out of the lab and onto the grid. The neighborhood is designed to be net-zero energy, and it's relatively close to NREL's Energy Systems Integration Facility, where our group is based.
What's more, Holy Cross Energy had been searching for a solution to manage the customer-owned energy resources and bulk generation in its system. In recent years, grid-connected, customer-owned resources have become much more affordable; Holy Cross's grid has been seeing 10 to 15 new rooftop solar installations per week. By 2030, the utility plans to install a 150-megawatt solar-powered summer peaking system. Meanwhile, though, the utility had to deal with nonstandardized devices causing instabilities on its grid, occasional outages from severe weather and wildfires, variable generation from solar and wind energy, and an uncertain market for rooftop solar and other energy generated by its customers.
In short, what Holy Cross was facing looked very much like what other grid operators are confronting throughout the country and much of the world.
To develop the AEG concept, our group is working at the union of two fields: optimization theory and control theory. Optimization theory finds solutions, but might ignore real-world conditions. Control algorithms work to stabilize a system under less-than-ideal conditions. Together these two fields form the theoretical scaffolding for an AEG.
Of course, this theoretical scaffolding has to conform to the messy constraints of the real world. For example, the controllers that run the AEG algorithms aren't supercomputers; they're common computer platforms or embedded controllers at the grid edge, and they have to complete their calculations in well under 1 second. That translates to simpler code, and in this case, simpler is better. Meanwhile, though, the calculations must factor in latency in communications; in a distributed network, there will still be time delays as signals travel from one node to the next. Our algorithms must also be able to operate with sparse or missing data, and contend with variations created by equipment from different vendors.
Even if we produce beautiful algorithms, their success still depends on the physics of the topology of power lines and the accuracy of the models of the devices. For a large commercial building, where you want to choose what to turn on and off, you need an accurate model of that building at the right timescales. If such a model doesn't exist, you have to build one. Doing that becomes an order of magnitude more difficult when the optimizations include many buildings and many models.
The Distributed Grid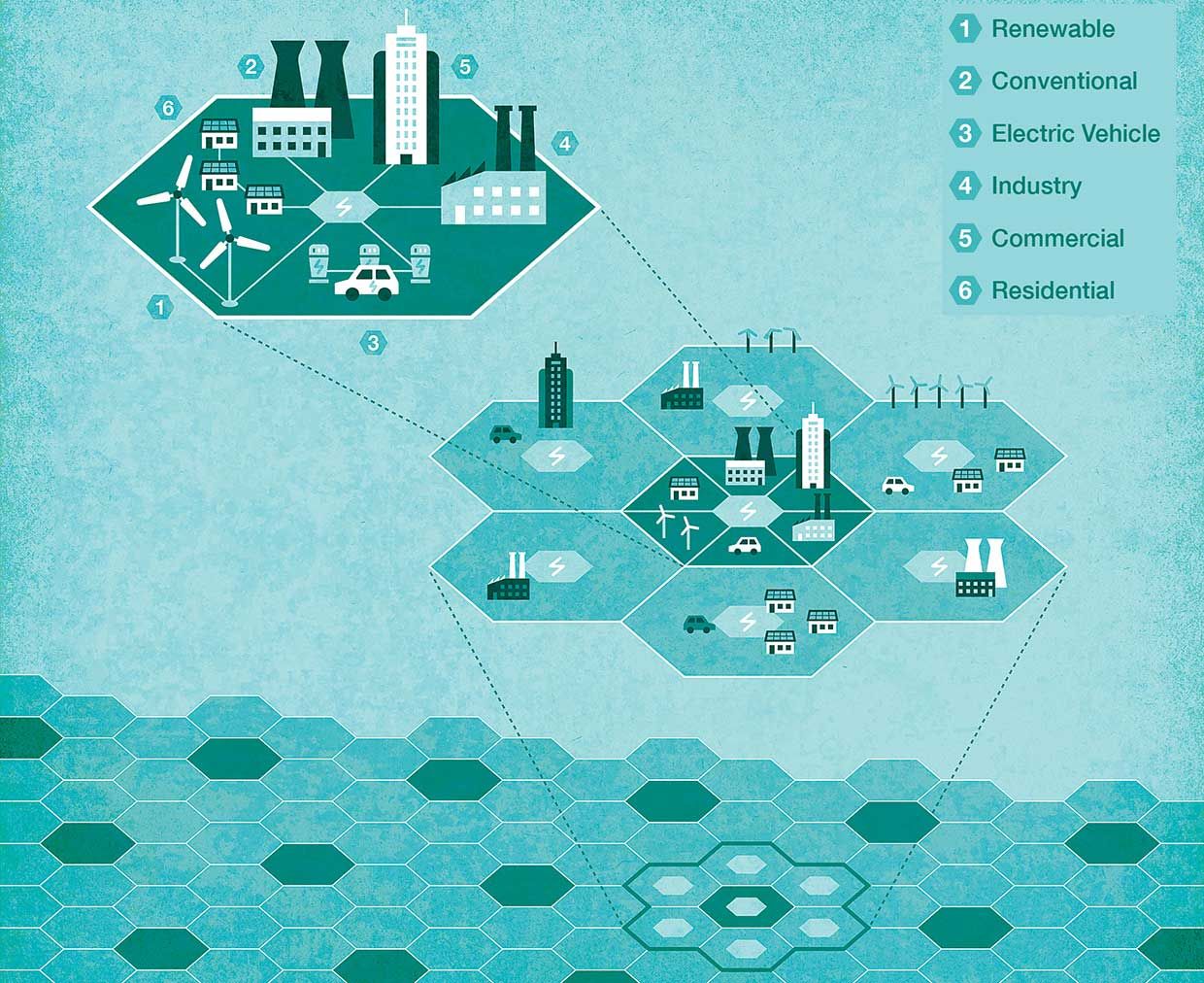
We've discovered that defining an abstract model is harder than optimizing the behavior of the real thing. In other words, we're cutting out the middleman" and instead using data and measurements to learn the optimal behavior directly. Using advanced data analytics and machine-learning techniques, we have dramatically sped up the time it takes to find optimal solutions.
To date, we've managed to overcome these hurdles at the small scale. NREL's Energy Systems Integration Facility is an advanced test bed for vetting new models of energy integration and power-grid modernization. We've been able to test how practical our algorithms are before deploying them in the field; they may look good on paper, but if you're trying to decide the fate of, say, a million devices in 1 second, you'd better be sure they really work. In our initial experiments with real power equipment-over 100 distributed resources at a time, totaling about half a megawatt-we were able to validate the AEG concepts by operating the systems across a range of scenarios.
Moving outside the laboratory, we first conducted a small demonstration in 2018 with the microgrid at the Stone Edge Farm Estate Vineyards and Winery in Sonoma, Calif., in partnership with the controller manufacturer Heila Technologies, in Somerville, Mass. The 785-kilowatt microgrid powers the 6.5-hectare farm through a combination of solar panels, fuel cells, and a microturbine that runs on natural gas and hydrogen, as well as storage in the form of batteries and hydrogen. An on-site electrolyzer feeds a hydrogen filling station for the farm's three fuel-cell electric cars.
The microgrid is connected to the main grid but can also operate independently in island" mode when needed. During wildfires in October 2017, for example, the main grid in and around Sonoma went down, and the farm was evacuated for 10 days, but the microgrid continued to run smoothly throughout. Our AEG demonstration at Stone Edge Farm connected 20 of the microgrid's power assets, and we showed how those assets could function collectively as a virtual power plant in a resilient and efficient way. This experiment served as another proof of concept for the AEG.
Basalt Vista is taking the AEG concept even further. A net-zero-energy affordable housing district developed by Habitat for Humanity for schoolteachers and other local workers, it already had a lot going for it. The final results of this real-world experiment aren't yet available, but seeing the first residents happily embrace this new frontier in energy has brought us another level of excitement about the future of AEGs.

At the National Renewable Energy Laboratory, researchers developed the optimization algorithms for the autonomous energy grid and tested them on real-world power systems [top], such as EV charging stations [bottom].
We engineered our early demonstrations so that other utilities could safely and easily run trials of the AEG approach using standard interoperability protocols. Now our group is considering the additional challenges that AEGs will face when we scale up and when we transition from Holy Cross Energy's rural deployment to the grid of a dense city. We're now studying what this idea will look like throughout an energy system-within a wind farm, inside an office building, on a factory complex-and what effects it will have on power transmission and distribution. We're also exploring the market mechanisms that would favor AEGs. It's clear that broad collaboration across disciplines will be needed to push the concept forward.
Our group at NREL isn't the only one looking at AEGs. Researchers at a number of leading universities have joined NREL in an effort to build the foundational science behind AEGs. Emiliano Dall'Anese of the University of Colorado, Boulder; Florian Dorfler of ETH Zurich; Ian A. Hiskens of the University of Michigan; Steven H. Low at Caltech's Netlab; and Sean Meyn of the University of Florida are early contributors to the AEG vision and have participated in a series of workshops on the topic. These collaborations are already producing dozens of technical papers each year that continue to build out the foundations for AEGs.
Within NREL, the circle of AEG contributors is also expanding, and we're looking at how the concept can apply to other forms of generation. One example is wind energy, where an AEG-enabled future means that control techniques similar to the ones deployed at Stone Edge Farm and Basalt Vista will autonomously manage large wind farms. By taking a large problem and breaking it into smaller cells, the AEG algorithms drastically reduce the time needed for all the turbines to come to a consensus on the wind's direction and respond by turning to face into the wind, which can boost the total energy production. Over the course of a year, that could mean millions of dollars of added revenue for the operator.
In our research, we're also considering how to optimally integrate the variable supply of wind energy into a bigger cell that includes other energy domains. For example, if a building's energy management system has access to wind forecasts, it could shift its load in real time to match the available wind power. During an afternoon lull in wind speed, the building's air-conditioning could be automatically adjusted upward a few degrees to reduce demand, with additional power drawn from battery storage.
We're also looking at communications infrastructure. To achieve the fast response required by an AEG cell, communications can't be clogged by simultaneous connections to millions of devices. In a new NREL partnership with the wireless company Anterix, of Woodland Park, N.J., we're demonstrating how a dedicated LTE network for device communications would operate.
Reliable operation, of course, assumes that communication channels are protected from cyberthreats and physical threats. The possibility of such attacks is guiding the conversation in power systems toward resilience and reliability. We believe that AEGs should minimize the impact of both deliberate attacks and natural disasters and make the grid more resilient. That's because the status of every grid-connected asset in every AEG cell will be checked on a second-by-second basis. Any sudden and unexpected change in status would trigger an appropriate response. In most cases, no drastic action would be required because the change is within the normal variability of operations. But if a major fault is the cause, the cell could automatically isolate itself, partially or entirely, from the rest of the network until the problem is resolved. Exploring the effects of AEGs on grid resilience is an ongoing priority at NREL.
For now, AEGs will show up first in neighborhoods like Basalt Vista and in other small-scale settings, such as hospitals and college campuses. Eventually, though, larger deployments should take place. In Hawaii, for instance, 350,000 customers have installed rooftop solar. With the state's mandate for 100 percent renewable power by 2045, the amount of distributed solar could triple. The utility, Hawaiian Electric Company, anticipates having to connect about 750,000 solar inverters, as well as battery systems, electric vehicles, and other distributed energy resources. Accordingly, HECO is looking to push autonomous control down to the local level as much as possible, to minimize the need for communication between the control center and each device. A completely autonomous grid will take some time to implement. In particular, we'll need to conduct extensive testing and demonstrations to show its feasibility with HECO's current communications and control infrastructures. But eventually the AEG concept will allow the utility to prioritize controls and focus on critical operations rather than trying to manage individual devices.
We think it will be another decade before AEG rollouts become commonplace, but an AEG market may arrive sooner. This past year we've made progress in commercializing the AEG algorithms, and with support from DOE's Solar Energy Technologies Office, NREL is now collaborating with Siemens on distributed control techniques. Likewise, NREL and the power management company Eaton Corp. have partnered to use the AEG work for autonomous, electrified transportation.
NREL has meanwhile explored how to sustain a distributed energy market using blockchain-based transactions-an option for so-called transactive energy markets. That project, in partnership with BlockCypher, successfully showed that a neighborhood like Basalt Vista could seamlessly monetize its energy sharing.
As we progress to a future of 100 percent clean energy, with a high concentration of inverter-based energy technologies, we will need a solution like AEGs to continue to operate the grid in a reliable, economic, and resilient way. Rather than looking to central power plants to meet their electricity needs, individual customers will increasingly be able to rely on one another. In a grid built on AEGs, being neighborly will be automatic.
This article appears in the December 2020 print issue as Good Grids Make Good Neighbors."
About the AuthorBenjamin Kroposki is an IEEE Fellow and director of the Power Systems Engineering Center at the National Renewable Energy Laboratory, in Golden, Colo. Andrey Bernstein is NREL's group manager of Energy Systems Control and Optimization, Jennifer King is a research engineer at NREL's National Wind Technology Center, and Fei Ding is a senior research engineer at NREL.